Collagen cross-linking plays a pivotal role in the strength and health of tissue scaffolds, influencing their mechanical properties and interactions with biomaterials. This article delves into the science behind collagen cross-linking, examining its significance in enhancing tissue strength and its implications for tissue engineering and drug delivery systems. By understanding the processes and effects of collagen cross-linking, we can better comprehend its critical function in the context of biomedical applications.
Key Takeaways
- Collagen cross-linking is essential for improving the mechanical properties of tissue scaffolds, particularly by integrating with materials like silica composites to address collagen's mechanical limitations and enhance structural integrity.
- The interaction between collagen and synthetic polymers, such as PDMS, is crucial for tissue engineering and drug delivery, affecting drug dissolution kinetics and the biological performance of scaffolds.
- Surface modifications of biomaterials can significantly alter protein adsorption, with implications for the design of tissue engineering scaffolds and drug delivery systems, ensuring biocompatibility and functionality.
The Role of Collagen Cross-Linking in Enhancing Tissue Scaffold Strength
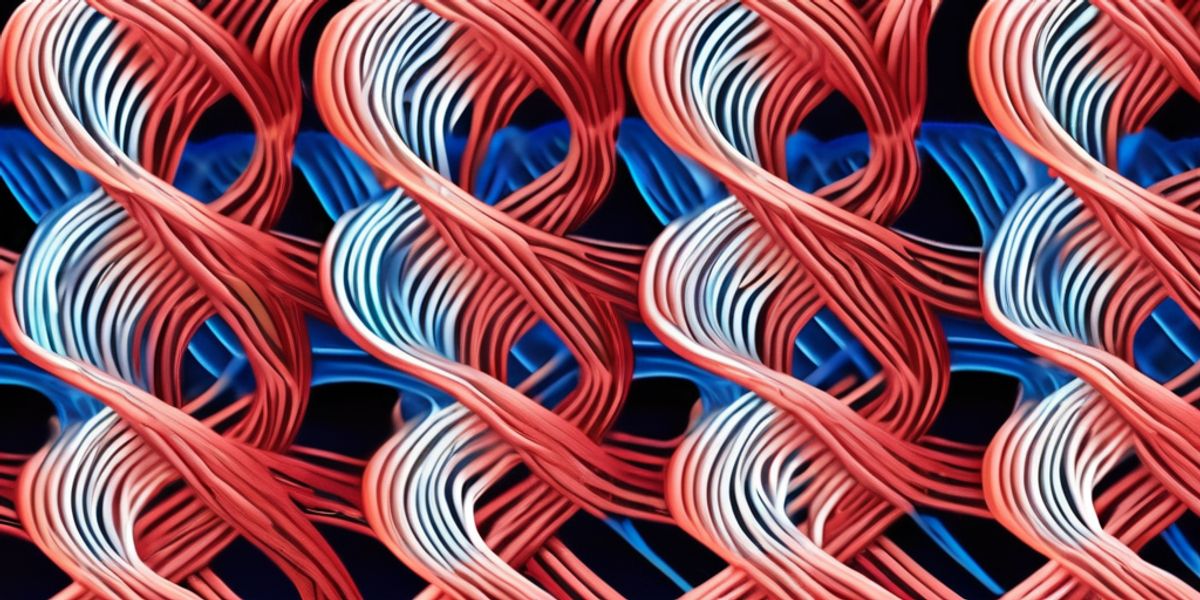
Understanding Collagen's Mechanical Limitations and Composite Integration
Collagen, while providing essential structural support in tissues, exhibits certain mechanical limitations that can be mitigated through integration with other materials. The tensile strength of collagen scaffolds is notably enhanced by incorporating non-collagenous materials such as silica composites or bioglass. This synergy not only improves mechanical properties but also preserves the natural structure of collagen, ensuring its functionality and compatibility with living tissues.
Enhancing the scaffold's mechanical attributes can be accomplished through intermolecular cross-linking, employing physical or chemical techniques to create a more robust tissue scaffold.
The stability of collagen scaffolds is challenged by exposure to water, which can lead to a reduction in structural integrity. Addressing this issue involves understanding the interaction dynamics between collagen and synthetic polymers, which is crucial for the design of biomaterials in tissue engineering and regenerative medicine. The following points highlight the key aspects of collagen composite integration:
- Integration with non-collagenous materials improves mechanical robustness.
- Maintaining collagen's natural structure is critical for cell growth and regeneration.
- Intermolecular cross-linking techniques enhance mechanical properties.
- Understanding the interaction with synthetic polymers aids in biomaterial design.
The Impact of Water on Collagen Scaffold Stability
The stability of collagen scaffolds is paramount in tissue engineering, where they serve as a matrix for cell growth and regeneration. However, the presence of water can significantly compromise the mechanical integrity of these scaffolds. Hydration, while essential for maintaining tissue health, can lead to the swelling and weakening of collagen structures. This is where creatine plays a supportive role. Creatine, known for its benefits in muscle and skeletal health, may also contribute to the stability of collagen by influencing cellular energy metabolism and potentially aiding in the maintenance of scaffold integrity.
Enhancing the scaffold's mechanical attributes can be accomplished through intermolecular cross-linking, which excludes water molecules and strengthens the scaffold.
To mitigate the effects of water on collagen scaffolds, various techniques have been developed. These include chemical cross-linking methods that reinforce the collagen matrix, making it more resistant to the degrading effects of water. The table below summarizes the impact of different cross-linking techniques on collagen scaffold stability:
Technique | Effect on Stability | Water Resistance |
---|---|---|
Physical | Moderate Increase | Low |
Chemical | High Increase | High |
Collagen's impact on skeletal and connective tissue disorders is significant in medical interventions, wound healing, and drug delivery mechanisms. Bioengineering advancements offer promising applications in cancer detection and treatment.
Techniques for Intermolecular Cross-Linking to Improve Mechanical Properties
The enhancement of collagen scaffolds is crucial for their application in tissue engineering. Physical and chemical cross-linking techniques are employed to address the mechanical limitations of collagen. By integrating collagen with non-collagenous materials such as silica composites or bioglass, the tensile strength and structural integrity of the scaffolds are significantly improved.
The exclusion of water molecules at the collagen-PDMS interface is a key factor in enhancing the structural integrity of the collagen, which is critical for maintaining the conformational rigidity necessary for tissue applications.
The use of phytic acid (PA) as a cross-linking agent has been shown to improve the mechanical properties and stability of decellularized tissues. This underscores the importance of selecting appropriate cross-linking agents and techniques to ensure the longevity and functionality of collagen-based biomaterials.
Here is a summary of common cross-linking agents and their effects:
- Phytic Acid (PA): Enhances mechanical properties and stability.
- Polydimethylsiloxane (PDMS): Optimizes interactions with collagen, improving scaffold performance.
- Silica Composites: Contribute to high porosity and surface area, beneficial for drug delivery.
The Significance of Surface Properties in Collagen-Polymer Interactions
The interaction between collagen and synthetic polymers such as polydimethylsiloxane (PDMS) is a cornerstone in the development of advanced biomaterials. Collagen's affinity for hydrophobic surfaces like PDMS is due to the hydrophobic interactions that stabilize its triple helix structure, enhancing the structural integrity of the collagen. This is crucial for maintaining the conformational rigidity necessary for its biological functions.
The exclusion of water molecules at the collagen-PDMS interface is a key factor in strengthening the hydrophobic bonds and preserving the collagen's natural structure.
Furthermore, the viscosity of PDMS can influence collagen binding, which is essential for tailoring composite properties to optimize biological performance. The implications of these interactions extend to drug delivery systems, where the adsorption of collagen onto drug carriers can significantly affect the dissolution kinetics of drugs.
It is also noteworthy that the time required for collagen to adhere to PDMS composites should be considered in the design of drug carriers. This ensures that the active substance is released at an optimal time, correlating with the amount of collagen adsorbed. Molecular dynamics simulations have shown that collagen exhibits a stronger affinity for hydrophobic surfaces compared to hydrophilic ones, which is a pivotal consideration in bioengineering applications.
Collagen and Biomaterial Interactions: Implications for Tissue Engineering and Drug Delivery
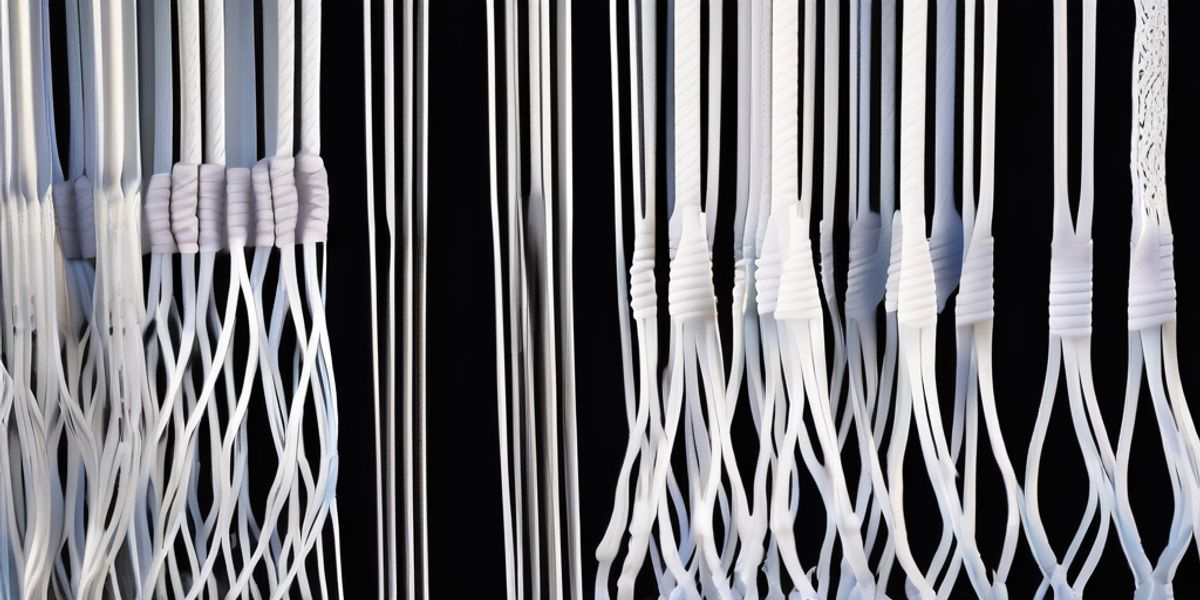
Collagen's Interaction with Silica Composites and Biocompatibility
The integration of collagen with silica composites, particularly those modified with polydimethylsiloxane (PDMS), represents a promising avenue in biomedical applications. The enhancement of collagen attachment to these composites is a pivotal step in creating materials that are not only structurally supportive but also biologically compatible with tissue scaffolds. Our research indicates that the inclusion of PDMS significantly improves the binding interactions between collagen and silica composites, while preserving the natural structure and functionality of collagen.
The biocompatibility of collagen, a material known for its excellent properties in tissue engineering, is further augmented when combined with PDMS-modified silica composites. This combination facilitates the creation of scaffolds that support cell growth and regeneration, essential for chronic skin wound treatment and other regenerative medicine applications. Moreover, the interaction dynamics between collagen and synthetic polymers like PDMS are crucial for the design of advanced biomaterials.
The ability to tailor the viscosity of PDMS in these composites opens new possibilities for optimizing biological performance. This fine-tuning is vital for achieving the desired drug release profiles and enhancing the bioavailability of therapeutics, especially in the context of drug delivery systems.
The implications of these findings extend to the field of drug delivery, where the adsorption of collagen onto drug carriers can significantly modify the dissolution kinetics of drugs. This interaction has the potential to revolutionize drug delivery strategies, making them more effective and targeted.
The Role of Collagen in Stimulating Cellular Responses for Regeneration
Collagen plays a pivotal role in regenerative medicine, acting as a molecular scaffold that supports cell growth and tissue repair. The interaction between collagen and PDMS-modified silica composites is particularly noteworthy, as it has significant implications for drug delivery and tissue engineering. Collagen's ability to maintain its natural structure when adhered to composites is essential for its functionality and compatibility with living tissues.
The advancements in collagen production and biotechnology have been instrumental in enhancing regenerative medicine. The integration of collagen with other materials, such as PDMS, has opened new avenues for creating scaffolds that not only support but also stimulate cellular responses for regeneration.
The study of collagen's interaction with synthetic polymers reveals the potential for designing biomaterials that can effectively support cell growth and regeneration. The following points highlight the importance of collagen in this context:
- Collagen's biocompatibility ensures its safe integration with other materials.
- The structural integrity of collagen is maintained upon binding, which is crucial for its biological functionality.
- Collagen scaffolds can stimulate platelet adhesion, which is likely to promote further cellular responses.
These insights into collagen's role in tissue scaffolds underscore the importance of ongoing research and development in the field of regenerative medicine. The supply chain for collagen emphasizes not only biocompatibility but also ethical practices, ensuring that advancements in the field are sustainable and responsible.
Investigating the Viscosity of PDMS in Collagen Binding and Scaffold Performance
The viscosity of polydimethylsiloxane (PDMS) plays a crucial role in the binding efficiency of collagen to silica composites, which is essential for scaffold performance in tissue engineering. Lower viscosity PDMS variants have shown a higher affinity for collagen adsorption, enhancing the composite's ability to support tissue regeneration.
Recent studies have demonstrated that PDMS with a viscosity of 25 centistokes (cSt) significantly improves collagen integration within the composite matrix. This finding is pivotal for the development of biomaterials that can effectively mimic the natural extracellular matrix and promote cellular responses conducive to healing.
The optimization of PDMS viscosity is a key factor in the design of collagen-based scaffolds, influencing not only the mechanical properties but also the biological interactions necessary for successful tissue engineering.
The following table summarizes the observed relationship between PDMS viscosity and collagen adsorption capacity:
PDMS Viscosity (cSt) | Collagen Adsorption Capacity |
---|---|
25 | High |
65 | Moderate |
750 | Low |
It is evident that as the PDMS viscosity increases, its ability to adsorb collagen decreases, which can inform the selection of PDMS types for specific biomedical applications.
Surface Modifications and Their Effects on Drug Dissolution Kinetics
Surface modifications of drug carriers, such as the incorporation of polydimethylsiloxanes (PDMS), play a pivotal role in the bioavailability and release profiles of therapeutic agents. The adsorption of proteins like collagen onto these modified carriers can significantly alter drug dissolution kinetics. This is particularly crucial for drugs that are sensitive to the protein matrix surrounding them, as it allows for a more targeted and effective delivery.
The interaction between drug carriers and the protein corona is a key factor in designing controlled release systems. By tailoring these interactions, it is possible to achieve a precise modulation of drug release, enhancing the therapeutic outcomes.
Electrolytes, while not directly involved in the surface modification process, are essential in maintaining the stability and function of biological systems. Their presence can influence the overall performance of drug delivery systems. For instance, the ionic strength of the surrounding medium can affect the protein adsorption on the carrier surface, which in turn impacts drug release kinetics.
The following table summarizes the effects of PDMS chain length on drug release from silica composites:
PDMS Chain Length | Drug Release Rate |
---|---|
Short | Faster |
Medium | Moderate |
Long | Slower |
Understanding these surface properties and their interactions with biological components like collagen is essential for the development of advanced drug delivery systems.
Conclusion
In summary, the exploration of collagen cross-linking reveals its pivotal role in enhancing tissue strength and health. The integration of collagen with non-collagenous materials such as silica composites and bioglass has been shown to improve mechanical properties, addressing collagen's limitations when exposed to water. Cross-linking techniques, both physical and chemical, are instrumental in reinforcing the mechanical attributes of collagen scaffolds, which is crucial for their application in tissue engineering and regenerative medicine. The interaction between collagen and synthetic polymers like PDMS-modified silica composites offers significant insights into drug delivery systems and the design of biomaterials. This study underscores the importance of maintaining the biological functionality of collagen when amalgamated with other substances, ensuring compatibility with living tissues for applications such as guided bone regeneration or wound healing. The findings from fluorescence microscopy and the observed adsorptive interactions between collagen and composite materials further support the potential of these enhanced scaffolds in biomedical applications.
Frequently Asked Questions
How does collagen cross-linking enhance tissue scaffold strength?
Collagen cross-linking enhances tissue scaffold strength by addressing collagen's mechanical limitations through integration with non-collagenous materials, such as silica composites or bioglass, to improve mechanical properties. Techniques like physical or chemical intermolecular cross-linking further enhance mechanical attributes, contributing to the structural integrity of the scaffolds.
What role does collagen play in tissue engineering and drug delivery?
In tissue engineering, collagen acts as a molecular scaffold that can stimulate cellular responses for regeneration. Collagen's interaction with materials like PDMS-modified silica composites is crucial for drug delivery, as it affects the dissolution kinetics of drugs and ensures the biocompatibility and functionality of biomaterials.
How do surface modifications affect collagen's interaction with biomaterials?
Surface modifications can significantly influence the interaction dynamics between collagen and synthetic polymers. For instance, the exclusion of water molecules at the collagen–PDMS interface strengthens hydrophobic bonds and maintains the conformational rigidity of collagen, which is essential for its biological functionality in applications such as guided bone regeneration or wound healing.