Cells are not merely passive entities within the body; they actively sense and respond to the mechanical properties of their surroundings. This article delves into the fascinating world of mechanotransduction, a process by which cells interpret and react to mechanical signals from the extracellular matrix (ECM), with a particular focus on the role of collagen. We explore how cells adapt to ECM stiffness and how advancements in artificial cell environments, such as ECM-like hydrogels, are enhancing our understanding of these complex cellular responses.
Key Takeaways
- Collagen and ECM stiffness are critical factors in mechanotransduction, influencing cell differentiation, migration, and resistance to treatments.
- Artificial cell environments, especially ECM-like hydrogels, provide a versatile platform for studying cellular responses to mechanical cues.
- Optimizing artificial environments such as 2D hydrogel substrates is essential for accurate in vitro studies of cellular mechanotransduction, including electrophysiological measurements.
Understanding Mechanotransduction: The Role of Collagen and ECM Stiffness
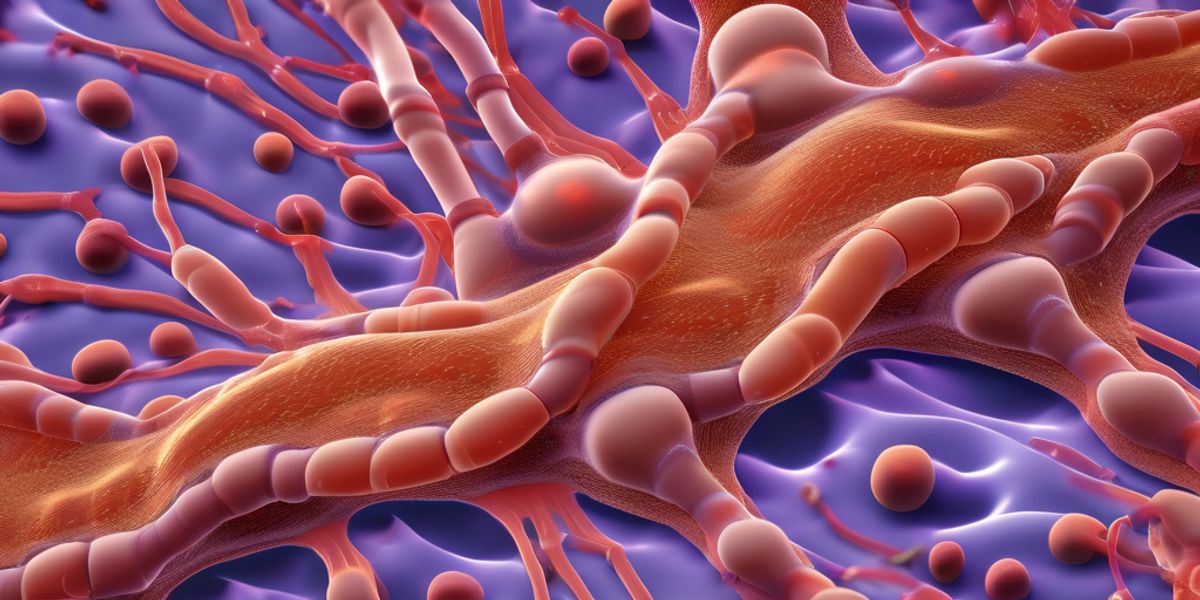
The Basics of Cellular Mechanosensing
Cells are equipped with the remarkable ability to sense and react to the mechanical properties of their surrounding extracellular matrix (ECM). This process, known as mechanotransduction, is crucial for a variety of cellular functions, including differentiation, migration, and response to external stimuli. ECM stiffness, in particular, plays a pivotal role in influencing cell behavior, dictating aspects such as cell spreading, polarity, and contractility.
The ECM is a complex network where collagen serves as a key structural protein, providing not only support but also mechanical cues to cells. Collagen's role extends to maintaining joint health, skin integrity, and the proper functioning of ligaments. It is also implicated in diseases like lupus. Enzymes such as lysyl oxidase and matrix metalloproteinases (MMPs), along with nutrients like manganese, are essential for collagen synthesis and maintaining tissue strength.
To study mechanotransduction effectively, researchers have developed artificial cell environments that mimic the ECM. These include hydrogels, flexible micropillars, and stretchable substrates, which offer variable mechanical properties and biocompatible chemical composition. Such advancements allow for precise control over the mechanical signals delivered to cells, paving the way for deeper insights into cellular responses.
The interplay between cellular mechanosensing and the ECM underscores the importance of creating environments that closely resemble natural tissue properties. This is vital for accurate in vitro studies and the development of therapeutic strategies.
Collagen's Influence on Cell Behavior
Collagen, a primary component of the extracellular matrix (ECM), plays a pivotal role in the cellular microenvironment. Collagen's interactions in drug delivery and regenerative medicine revolutionize strategies, supporting cell growth and tissue repair. Advancements in production and biotechnology enhance regenerative medicine responsibly. Continuous use of collagen supplementation has been shown to benefit both the internal and external layers of the skin. Externally, it hydrates skin cells, improving elasticity and density. Internally, it bolsters the function of the dermis, the living tissue beneath the skin's surface.
Collagen's regulatory effect on fibroblast function is a key factor in redefining our perspective on skin aging. It influences cell spreading, polarity, and contractility, which are essential aspects of mechanotransduction.
The table below summarizes the effects of collagen on various cellular processes:
Cellular Process | Effect of Collagen |
---|---|
Cell Growth | Supports |
Tissue Repair | Enhances |
Skin Hydration | Improves |
Skin Elasticity | Increases |
Dermis Function | Strengthens |
Understanding these effects is crucial for developing artificial cell environments that mimic the natural ECM, providing a more accurate platform for studying cell behavior and mechanotransduction.
ECM Stiffness and Its Effects on Cell Dynamics
The extracellular matrix (ECM) is not just a passive structure surrounding cells; it plays an active role in regulating cellular behaviors through mechanotransduction. ECM stiffness is a critical factor that influences cell dynamics, including aspects such as cell spreading, polarity, and contractility. The ability of cells to sense and respond to the mechanical properties of the ECM is essential for various physiological processes.
Artificial cell environments aim to mimic these natural conditions by offering variable mechanical properties and biocompatible chemical compositions. These environments are designed to be adaptable for different cell types and conditions, providing a more accurate representation of how cells interact with their surroundings in vivo. Among the various approaches to simulate mechanical cues, ECM-like hydrogels have emerged as one of the most effective due to their three-dimensional polymer networks that closely resemble natural ECM.
The development of artificial environments that accurately reflect the ECM's mechanical properties is crucial for advancing our understanding of cell behavior and the underlying mechanisms of mechanotransduction.
Here is a summary of the effects of ECM stiffness on cell dynamics:
- Cell Spreading: Cells tend to spread more on stiffer substrates, which can affect their growth and function.
- Cell Polarity: The orientation and structure of cells are influenced by the stiffness of the ECM, impacting processes like migration.
- Cell Contractility: The ability of cells to contract and generate tension is modulated by the stiffness of the ECM, which can affect their interaction with the matrix and other cells.
These insights pave the way for the next section, which delves into the advancements in artificial cell environments and how they contribute to the field of mechanotransduction research.
Advancements in Artificial Cell Environments
The quest to replicate the intricate dance of cells within their natural habitat has led to significant advancements in artificial cell environments. These novel platforms enable precise control over mechanical and chemical cues, mimicking the extracellular matrix (ECM) and influencing cellular behavior in unprecedented ways.
The development of ECM-like hydrogels represents a leap forward in our ability to study mechanotransduction. These 3D polymer networks offer high water retention and can be engineered to closely resemble the ECM, providing a more physiologically relevant context for cell culture.
Innovative materials such as flexible PDMS micropillars, flow chambers, and stretchable substrates have emerged, each with their unique ability to convey mechanical signals to cells. Below is a list of some key approaches:
- Flexible PDMS micropillars for studying cell traction forces
- Flow chambers to simulate shear stress
- Stretchable substrates to apply uniaxial or biaxial strain
These technologies not only enhance our understanding of cell mechanics but also hold the potential to revolutionize cell culture methods, paving the way for more accurate models of tissue behavior and disease progression.
Exploring ECM-like Hydrogels for Mechanotransduction Studies
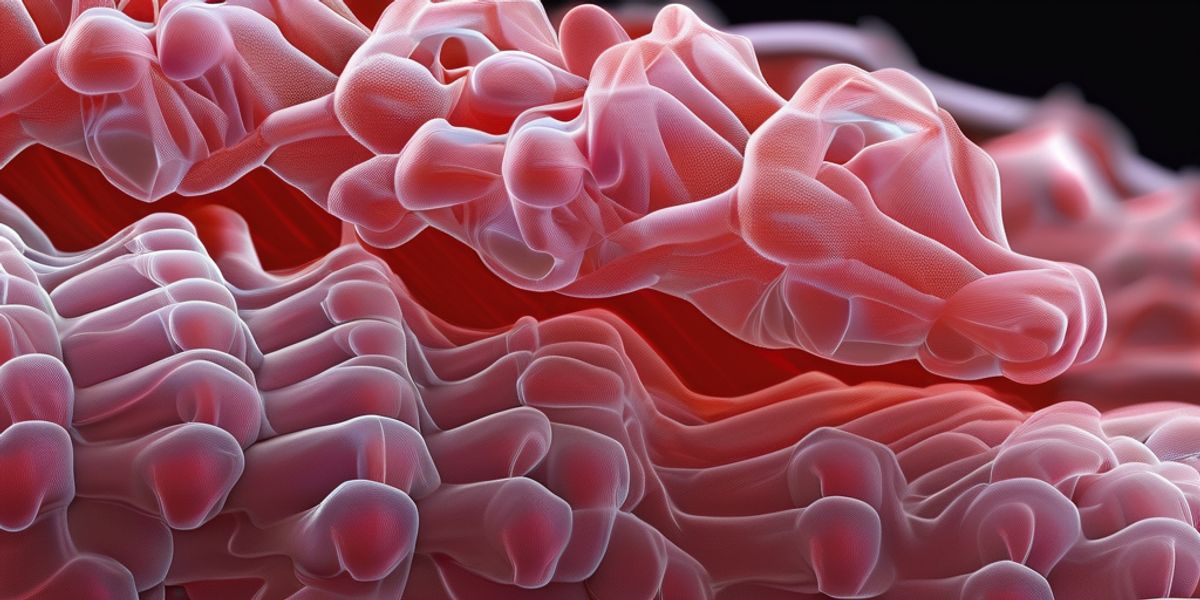
Characterizing Hydrogel Systems for Cellular Response
Hydrogel systems are pivotal in the study of cellular mechanotransduction due to their ability to mimic the extracellular matrix (ECM). Characterizing these hydrogels is essential to understand how cells respond to mechanical signals. The characterization process varies depending on whether the hydrogel is used in a 2D or 3D culture system. In 2D, the focus is on surface properties that affect cell growth, while in 3D, the emphasis shifts to properties that influence cell encapsulation and survival.
For 3D cultures, the diffusion of molecules such as nutrients and oxygen through the hydrogel is critical for cell viability. This is particularly important as encapsulated cells do not have direct access to the medium. Additionally, the mechanism of cell adhesion, involving cell surface receptors, is a key factor in the characterization of hydrogels.
The viscoelastic properties of hydrogels can significantly impact the behavior of cells, such as fibroblasts, which are sensitive to the mechanical environment. Hydrogels with optimized properties can lead to notable changes in cell structure and function, highlighting the importance of precise characterization.
The table below summarizes key properties of hydrogels that are crucial for 3D cell culture applications:
Property | Importance |
---|---|
Medium Diffusion | Ensures nutrient and oxygen supply |
Oxygen Diffusion | Critical for cellular respiration |
Cell Adhesion Mechanism | Influences cell survival and function |
Mechanical Properties | Affects cell morphology and signaling |
Hydration is a fundamental aspect of hydrogel systems, influencing their swelling behavior and, consequently, the diffusion capabilities. Proper hydration ensures that the hydrogel maintains a balance between stiffness and flexibility, which is vital for the accurate simulation of the ECM and the study of mechanotransduction.
Viscoelastic Properties and Their Impact on Fibroblasts
The viscoelastic properties of ECM-like hydrogels are pivotal in dictating fibroblast behavior. Fibroblasts respond to the mechanical cues of their environment, adapting their morphology and function accordingly. This adaptability is crucial for maintaining the dynamic balance of the ECM, which is essential for skin health and combating signs of aging.
The viscoelasticity of hydrogels can be tuned to mimic the natural ECM, providing a versatile platform for studying fibroblast responses under various mechanical conditions.
In the context of skin aging, the relationship between the viscoelastic properties of the ECM and human dermal fibroblasts (HDFs) is highly correlated. A disrupted ECM balance often leads to an aged fibroblast phenotype, characterized by a loss of cellular dynamics and a reduced ability to secrete new ECM components. By utilizing hydrogels with tailored viscoelastic properties, researchers can better understand and potentially counteract these aging processes.
The following table summarizes key findings from a study on the impact of viscoelastic properties on fibroblast behavior:
ECM Component | Function | Impact on Fibroblasts |
---|---|---|
FN-1 | Supports cytoskeleton | Enhances migration and proliferation |
LM-5 | Component of basement membrane | Stimulates adhesion and movement |
Vimentin | Maintains cellular integrity | Preserves contraction function |
These components are integral to the structural and mechanical integrity of fibroblasts, and their presence in hydrogels can significantly influence cellular responses.
Optimizing 2D Substrates for Electrophysiological Measurements
The optimization of 2D hydrogel substrates for electrophysiological measurements is a pivotal step in advancing our understanding of cellular mechanotransduction. These substrates provide a more physiologically relevant environment for cardiac cells, such as the HL-1 cell line, enhancing the accuracy of electrophysiological data.
The use of ECM-like hydrogels as 2D substrates has shown promising results in mimicking the natural cellular milieu, thereby offering a refined platform for studying the intricate processes of cell signaling and behavior.
Electrolytes play a crucial role in maintaining the balance of the extracellular environment, ensuring that cells function optimally during electrophysiological studies. The incorporation of creatine in the hydrogel matrix has been observed to support cellular energy metabolism, which is essential for the sustained activity of excitable cells like neurons and cardiomyocytes.
- **Key Advantages of Optimized 2D Hydrogel Substrates: **
- Enhanced cell adhesion and proliferation
- Improved simulation of the natural ECM
- Adjustable mechanical and structural properties
- Cost-effective and suitable for various cell types
The development of these substrates is a testament to the ingenuity of biomaterials research, providing a versatile tool for scientists to probe the complexities of cellular responses to mechanical stimuli.
The Future of Hydrogel Applications in Cell Culture
The versatility of hydrogels in cell culture applications is becoming increasingly evident. As we look to the future, the integration of growth factors and glycosaminoglycans (GAGs) into hydrogel matrices is anticipated to enhance tissue support and mimic the natural cellular environment more closely. This integration could lead to significant advancements in tissue engineering and regenerative medicine.
Hydrogels have shown promise in providing a three-dimensional scaffold that supports cell growth and differentiation. Their ability to be tailored for specific mechanical and biochemical properties allows for the creation of customized environments that can influence cell behavior in predictable ways. For instance, the incorporation of hydrolyzed collagen into hydrogels can improve cell adhesion and proliferation, while maintaining hydration and ensuring proper nutrient diffusion.
The potential of hydrogels to revolutionize cell culture lies in their capacity to simulate the extracellular matrix, providing a dynamic and physiologically relevant platform for studying cell mechanics and responses.
Future research will likely focus on optimizing hydrogel systems to reduce immunogenicity and improve integrin-mediated signaling pathways. This will enable a more accurate simulation of the cellular microenvironment and facilitate the study of complex cell interactions. The table below summarizes the key properties of hydrogels that are critical for cell culture applications:
Property | Importance for Cell Culture |
---|---|
Swelling Capacity | Ensures adequate hydration and space for cell expansion |
Oxygen Diffusion | Allows for proper cellular respiration |
Mechanical Properties | Influences cell morphology and function |
Degradation Rate | Controls scaffold longevity and cell turnover |
As we continue to refine hydrogel technology, the goal is to create systems that not only support basic cell culture but also enable sophisticated models for disease research, drug discovery, and ultimately, the development of personalized medicine.
Conclusion
In summary, the intricate dance of mechanotransduction within the cellular microenvironment underscores the profound influence of the extracellular matrix (ECM) on cell behavior. This article has highlighted the critical role of ECM stiffness and composition in modulating cellular functions such as migration, differentiation, and response to therapeutic interventions. The development of ECM-like hydrogels and other biomimetic substrates has opened new avenues for studying cellular responses in more physiologically relevant conditions. These advancements not only enhance our understanding of cell biology but also pave the way for innovative approaches in tissue engineering and regenerative medicine. As we continue to unravel the complexities of mechanotransduction, it is clear that the ECM is not merely a scaffold but a dynamic participant in cellular communication and function.
Frequently Asked Questions
What is mechanotransduction and how does collagen play a role?
Mechanotransduction refers to the process by which cells sense and respond to mechanical signals from their environment. Collagen, a key component of the extracellular matrix (ECM), can influence cell behavior by altering mechanical properties such as stiffness, which affects processes like cell differentiation, migration, and resistance to chemotherapy.
Why are ECM-like hydrogels important for studying mechanotransduction?
ECM-like hydrogels are important because they provide a biocompatible environment that mimics the natural extracellular matrix, allowing researchers to study how cells respond to various mechanical cues. These hydrogels can be engineered with adjustable mechanical and structural properties, making them ideal for observing cellular responses in both 2D and 3D cultures.
How do substrate properties like stiffness impact cell behavior?
Substrate properties such as stiffness have a significant impact on cell behavior. Stiffer substrates can promote cell spreading, polarity, and contractility, while softer substrates may lead to different cellular responses. This interaction is a key aspect of mechanotransduction, as cells adjust their functions in response to the rigidity of their surrounding environment.